Demonstrations
[MUSIC]
Hello. Welcome back to another episode of
Undercooled and
Materials Education podcast.
Today, Tim and I are going
to talk about demonstrations,
mostly because this was Tim's life for
quite some time before
he became a lecturer.
Sure was.
Let's start with having Tim tell you what
he used to do. Go ahead, Tim.
Sure. Once upon a time when I started at
MSE, my first job in MSE
was being the support guy.
So part of that was helping out with lab
classes. But another big part of it was
developing and implementing the classroom
demos for mostly for our intro classes,
a couple advanced classes as well. So it
was a great learning experience for me.
Hey, what is all this stuff? It was a
good way to get into the field. But
working with different
faculty to come up with ideas for demos
that they wanted to do in
their classes, then trying to help
make those real was a really interesting
bit of work that I did and
something I would definitely
recommend to anyone who wants to figure
out a little bit more
on the teaching side.
Great. So why demos? What do you think
the value of the demonstration is?
You know, I could see three main
arguments in favor of having classroom
demos. One of them is
engagement. They're fun. That's in its
own right. There is some
value to that. That's not going to
be enough by itself. But sometimes you
just want to say, hey,
let's do something different and
interesting to bring the students back to
life a little bit. So
that's fine. And for that, almost
anything works. The second aspect and the
one that I'd argue is the
most important out of these three
is the fact that you can have students
make and test predictions in
real time live while they're
learning the content and to have a much
faster, much faster
feedback response than what you get
in a real lab when you're doing
experiments or when you're doing
simulations. You know, that can be a
year's effort to find out whether your
prediction was correct.
So to find out in minutes,
do I understand this? Am I thinking about
it correctly? That's very powerful.
And then the third aspect is that demos
can be used as a launching point for a
further investigation
on the student side. So that can be a way
to develop into an out of
class assignment, like a
homework, for example. And that can be a
nice way to say, okay, we're learning
this stuff in class,
but now go out and connect it to the rest
of the world. That's
pretty good. So of course,
I've been teaching intro to materials for
a very long time, and I
don't think I could do it without
doing demonstrations. Otherwise, it's
just us talking, you know,
yap, yap, yap in front of the
class, and they don't really believe that
anything I'm saying is
true. So at least the demos show
that there's some real life thing going
on. And I tend to far
prefer physical demonstrations
to all the virtual demonstrations that
have been coming up. I've
seen a lot of them. And I,
you know, in my opinion, I just don't get
it. They seem like very black box
modules, where you have
little sliders to change parameters, and
you just look how the math
changes. And that just kind of
leaves me kind of flat. And there's no
connection to the real
physical world when you do that.
Whereas when you try a real physical
demo, you know, you eat
something up, you stretch something,
you bang on it, you do something to it,
you actually see it
respond. And it's hard to deny
that that's a real thing that just
happened. And so to me, physical
demonstrations are so powerful,
besides all the three things you just
mentioned, I think they're
just powerful because they
expand a student's everyday experience.
And I don't think everyday
experience should ever be
discounted. It's what we are, you know,
we grow up, we fall down and
it hurts, we run into a wall and
it hurts. And it's a real thing to
anybody who's experienced any of those
things. And unfortunately,
everyday experience doesn't always serve
us well. And that's kind of our job as
educators to explain
to students that, yeah, you know a lot,
and we really value what you
know. But there's a lot you
probably don't know. A lot of things you
haven't quite experienced
that we want to make you aware
of. Because if you can understand these
concepts and these ideas that we're
trying to teach you,
and start to believe it and start to fold
it into your everyday
experience, you're going to develop
a really high quality intuition that will
help you design things using the
fundamentals of material
science. It's kind of what we do. And so
doing demonstrations, I
think is just a critical part
of teaching material science. So at least
that's how I feel. I
never cared much for these,
the physics FET demonstrations or
JavaScript things because,
yeah, they're kind of cool, but
they illustrate certain things, but they
don't really add to that
individual experience. And I
think that's really special. Yeah, I
definitely have to agree on how important
students' individual
experiences are, both what they bring to
the class as well as what
they experience in class.
And this is where the right demo at the
right time can be so
powerful because of students from
their everyday life and from their prior
experience, if they have one expectation
about how a material
should behave and then they see it in
real time live in front of their eyes
behaving in a different
way, that's not what they expected. That
can be a really powerful
catalyst to get them to
evaluate how they understand the content
that we're trying to teach.
Right. Now, of course, the kind of demo
that you would choose
depends pretty much entirely on who
the audience is. So, you know, we've kind
of just been talking about
intro to materials courses,
because that's what you and I do the most
of the time. But there's a
lot of other audiences that
I think you have to tune the demo to the
audience to make sure they'll
be able to receive it and get
something out of it. You know, for
instance, when you go into a K through
six class doing outreach,
you're probably not going to show them
the zinc aluminum system
where you get this metastable
state and you smack it and it gets hot.
They would have no chance
of really understanding that.
On the other hand, liquid nitrogen ice
cream. Wow. That's a fun
thing for little kids to do. And
it connects with their everyday
experience with eating ice cream. And now
you can talk to them
about how you can change the texture of
that ice cream by changing
the kinetics of that experiment,
which is kind of cool. I love that
example, because that is
one that really does work at
all levels exactly as you described, even
for a young child, it's
ice cream. But then that can
go all the way up to an undergraduate or
even a graduate kinetics
course where you're looking at
the nucleation and growth of ice crystals
and how this is affected by
the cooling rate and by the
ratio of, you know, nitrogen to cream and
these other factors, it can
become very complex or not
as you want it to be. And that's
something that I think we should try to
aim for with all of our
demos is that they really do work at
multiple levels so that
we're not trying to create
entirely new suites of content for every
separate audience. And that's
great if we can pull it off,
but sometimes it's hard. You know, so
like a silly putty. Yeah, we do use that
to show viscoelastic
behavior at any level, but it's a really
simple concept, at least the
silly putty part. So that's
more amenable to K through six or high
school students, even
intro material students. But I
don't think we want to be pulling out
silly putty for a graduate course and
polymers. I think they
know that. But the other kinds of demos
that we do all the time, a lot of us go
into high schools to
try to get students excited about
material science so that they might
choose a materials career.
And so I know you've done a lot of this.
I've done some. And I think the most
exciting tool that we
can bring to high schools is the portable
scanning electron
microscopes. Maybe you can talk a little
bit about how we do that. Sure. The
future is an amazing place. You can just
take an SEM and carry
it around in the back of your car now.
And there are tabletop models made by a
variety of manufacturers
now that we've taken to classrooms, to
museums, to public
science centers even. And
you know, the power of that, this will
also connect back to your point about
virtual demos, I think.
One of the things that really makes
materials science special
is how we work across so many
length scales. And to be able to zoom in
real time from 10x to 100
to 1000 to 10,000 to 50,000,
and to see a single object at so many
different length scales
can really be a mind-blowing
experience and get people to think, "I
didn't even know that
existed." So I am very glad that
we have the ability technologically to do
that now and to bring that to people
instead of having to wait until they're
already in the university.
Especially when you show them things like
shark skin, because they
hear about the Olympics and
athletes wearing these shark skin bathing
suits for swimming, which
are outlawed now because they
work too well. But to show them what
shark skin actually
looks like, to show them how
butterfly wings are really diffraction
gratings, and that's why
you get all those weird colors
off the wings when they shimmer. Even
just showing them the width of Lincoln's
leg on a Lincoln penny,
because his leg is 100 microns wide, and
you can just barely see
that on the penny with your
naked eye, but you can really see it in
the SEM. And it just gives a way to
connect with real life
at all these different length scales. And
I think that's really
important for high school kids,
because they haven't really appreciated
that yet. Whereas college
kids and certainly graduate
students, they have. They understand all
that. But you can use the
same tool to do other things
for the graduate students, looking at
twinning and all this other neat stuff.
And then the other place we use
demonstrations is when we go to career
fairs or majors fairs,
where we bring things to try to get
people to come to our
table to come to talk to us.
So we have a shot at convincing them how
cool material science
is. And those are a little
different. I mean, I think the demo I've
always liked the most is
the one with the CD that you
squish in there, you spray that stuff, it
slams out. Things that
blow up or explode really get
the students excited. Maybe as soon as
there's a crowd, a crowd of
people with everyone wearing
safety glasses and loud noises happening,
and the whole room wants to
know, well, what's happening
over there? That must be the cool kids
table. So it can certainly be
a good way to rope people in.
That particular demo, how it works is you
have to acquire these
legendary artifacts known as
compact discs, they come from a previous
age. And if you can get
enough of them in one place,
what you do is you put the CD under some
flexural stress. So have a
mount that you can clamp it into
and squeeze it a little bit and spray it
with furniture polish.
And what will happen is that
the furniture polish will change the
cross linking between the
polycarbonate chains in the CD,
and it will reach the point where the
strength of the polycarbonate
changes, but it's still held
under this constant stress and eventually
it fails. And so the CD
will snap and jump out of
the holder into usually a few pieces. And
it's a really great way
to illustrate that chemical
stresses and chemical processing of
materials alongside mechanical stresses
are really important
to understanding processing and materials
properties. Yep,
that's a cool one. And then
finally, another really important kind of
demonstration for the materials community
is what we do with our teachers camps.
And you've been involved with the
teachers camps for years,
Tim, and maybe you can talk about, they
have a different set of
boundary conditions, right?
Things have to be cheap and they have to
fit into lesson plans. And
that's been pretty much the
hallmark of the whole ASM Foundation
teachers camps is showing
these teachers how to do these
demonstrations in class as part of the
curriculum. And so maybe you can describe
some of those for us.
Yeah, the ASM teachers camp, anyone out
there who's listening to
this, if there is one in your area
and you haven't interacted with it, I
would absolutely recommend
that. It's a great experience.
It is a good way to build relationships
within your community as
well. And also a good way to
pick up some good tips for your own MSE
classes. Here's a couple
examples. Many of them are quite
chemistry oriented. A lot of the schools
where these teachers are
teaching don't have a material
science class. They might have a
"engineering course." Some
have physics classes, but mostly
they're teaching chemistry. And so
they're approaching really material
science from the view
of what can a high school chemistry
student engage with. So they're looking
at crystal growth and
single replacement reactions. For
example, there is a demo that they do
where students take a
galvanized nail. They immerse it in a
copper, I believe it's a
copper chloride solution, and they
grow metallic copper off of this nail as
the copper replaces the zinc atoms on the
surface of the nail.
So that's a really great one. And another
example that we use all the
time at the college level as
college level is the iron wire. It's
truly one of the classic greats of MSE
demos, I feel. And Steve,
I know you use the iron wire in your
classes as well. So can
you talk us through that one?
Yeah, I love that one. This is of course
where you take a piano
wire, which is a very,
very low carbon steel, almost zero
percent carbon, and you stretch it out
and you do something you
tell the kids, "Don't do this at home."
And you take the two ends
and you plug it into a variac
and you just crank it up. And as the wire
gets hotter and higher,
of course you get thermal
expansion. But it's two effects that
you're trying to teach.
One is thermal expansion,
of course the other is the BCC to FCC
phase transformation of
iron. And so as it's heating,
it starts to get hot, red hot, and it's
sagging. But right when it hits the
transition to FCC iron,
because FCC is more close packed than
BCC, it's a volumetric phase
transformation. So it shrinks.
So it goes down, then it goes up, and
then because you're still
heating it, it eventually
expands and goes down. And it's really
obvious when you cool it.
So once it's glowing hot,
all the way down above, it's an
austenitic material, you just turn off
the variac and it comes back up
and then it drops down and then it goes
back up again. And most
students get the thermal expansion
part, but very, very few remember about
the volumetric phase
transformation, because that's
kind of a new concept for most students.
Most students, they come
into college and they've
heard of phase transformations. They know
you can go from liquid to
solid to gas, and that's it.
That's all you learn in high school. And
now we tell them that a
phase can be different
crystal structures. And that is kind of
very disturbing to them
because it's challenging what
they thought they understood. And now we
tell them that there's
more to it. And so what is a
different crystal structure? Well, there
are, you know, you can show them x-ray
diffraction patterns,
but I don't know if they're going to
believe that. But if you show them a
volumetric change and how
it's reversible, it goes up and down. And
then I tell them how when
I was a graduate student,
I used single crystal iron samples that
had to be very expensive
because you can only make them
with something called strain annealing.
So my little tiny sample
was like 800 bucks. And once
I made the mistake when I was sputter
cleaning it, you know, I had
two wires spot welded to it,
right? So I would heat the wires and it
would get hot. Well, I took
it up too high. I took it up
above the BCC and FCC phase
transformation. And yeah, it came back as
BCC, but it wasn't a single
crystal anymore. It was a mess. And my
advisor was very kind to me and said,
don't worry about it.
Just don't do it again. And he bought me
a new one. That's very nice.
But it's, you know, you get,
you know, those of us who do these things
in research, we get burned
all the time by our demos,
which brings up a really interesting
point about demos. You kind of have to
have a sense of humor,
because they don't always work. In fact,
it's very easy to have them not work.
Yes, they will betray you when you need
them the most. Right. And a
lot of that has to do with
the person who sources the materials,
puts it together, trains
the graduate student that's
going to run the demonstration. And often
the only way for that
person, namely you, to really
know that you have a handle on this was
for you to actually come to
class and do the demo yourself.
So you felt extremely comfortable that it
would work. Then you
would be in a position to show
somebody how to do it. For example, the
iron thing, it doesn't always work,
right? And it doesn't
always work. Because if you use one piece
of wire too many times, you
get all these green boundaries
and nano crystalline material, and it
just messes everything up, because those
green boundaries pin
the transformations. And so the trick for
that is to always
start with a fresh wire.
And so, you know, where do you learn
that? Well, by experience,
by doing it a few dozen times and seeing
when it works and when it
doesn't, and finding out what
the stopping points are another one, I
use this to get graduate
students all the time, because
another factor is how quickly you turn up
the temperature on the
wire, because you're feeding
at current, that's your heating mechanism
is dual heating. And the
resistance is a function
of the temperature. But often a novice
implementer of this demo won't be
considering the fact that
the resistance of the wire is changing.
And so they crank up the
current, and then it blows the
fuse in the variac, and then nothing
happens at all. And I did everything
right, what went wrong?
Well, you forgot that the resistance is
lower when it's colder, and
you fed it too much current,
and the fuse couldn't handle it. But if
you ramp it up slowly and let that
resistance go up as well,
then so there are so many little
subtleties like that that, yeah, you
should if you're bringing a
new demo into your class, I would no
kidding recommend
practicing at least a dozen times
before doing it in front of the students
if you want to have a
good chance of success.
If you want to model is how to handle
things going wrong, oh, yeah, jump right
in and give it a go,
something will go wrong for sure. But
usually we want more than that.
Another favorite demo when you want to
show strengthening of
metals, you take a pretty much
pure aluminum bar, and you anneal it. And
then when you anneal it
pure aluminum is very soft,
you can bend that bar very easily. And
then hopefully when you
bend it, you've introduced
enough dislocations that it's very
difficult to bend it back. And so it
sounds great in principle.
But I can't tell you how many times that
demo hasn't worked.
Either the bar wasn't annealed
enough. And I could barely bend it to
begin with. You'd like to do this by
asking someone who thinks
they're really weak, but would like to
demonstrate they can bend metal, they
have them come up. And
if they can't bend it, oh my god, it just
ruins the whole thing. Yeah.
And but the other problem is
if it's too annealed, yeah, it's easy to
bend. But then the strong
people in the class can actually
bend it back. And that again, takes your
thunder away because you
want it. I mean, I'll never
forget. I had one bar that was perfect.
This very weak individual
was able to just bend it.
It made them feel great. And then I said,
anyone think they're a
really big strong guy,
you know, guy or girl, and this Marine
came up. Oh boy. And he
just, he couldn't bend it back.
And I thought he was going to kill me. I
thought he was going to,
because he was so embarrassed
that he couldn't bend back and he was a
Marine. And so I was like a little
fearful, but that was
exactly what you want to happen. So how
do you hit that just right?
And the only way to really do
that is to have enough bars so you can
try one. And so if I've
learned anything about demos,
it's all the things that can go wrong
with a demo and it's going
to happen. And you're just
going to have to explain to the students
that this is real life,
you know, it's complicated
and lots of different things happen. And,
but luckily the
something you said earlier is so
true. What really is important is that
students make a prediction
before you do the demo. And I
believe Eric Mazur has written some
education research papers
demonstrating this and showing
that if the students can predict what's
going to happen before it
happens, it doesn't matter if
they get it right or wrong because
they're roped in. Once they've made a
prediction, they've got
skin in the game. They want to know
what's going to happen. They
want to see if they're right or
wrong. And so they end up paying much
more attention and then
they want to understand why.
And so as long as you can produce a good
reason why, even if the
demo doesn't work, if you can
tell them why it didn't work, they'll
actually learn a lot. And
ultimately our job isn't to make
the demos go great. Sometimes it's good
they see that we fail all the time
because that's normal,
not the other way around. But what really
matters is that they
learn the concept and bring
something into their everyday experience
that they didn't have before
to give them the ability to
think about how more complex things might
happen in the future. I
couldn't agree more. What are
some other greatest hits that we have?
We've talked about a few metals ones. Oh,
a lot of polymer ones.
Yeah. I know one polymer demo that I
really like because it also brings in
functional properties
a little bit is making polarizers. That's
something that's very low
cost, very easy to do, and looks
really cool, but also reminds students,
"Hey, there's more to life
than strength and Young's
modulus and things like that." Right. So
which one do you like? So I've
tried it a couple of different
ways. I've found the best results with
garbage bags actually.
Polyethylene garbage bags. Yes,
polyethylene garbage bags to be clear.
Well, also to be clear. But the trick
with those is getting
the strain rate right so that you can
strain them far enough to
get some good alignment of the
polymer chains to build in that
anisotropy. So they become polarizing
without actually tearing
them. And that can require a little bit
of a delicate touch, but
it's one of those things
you practice it. And as soon as you get
it right the first time, you
say, "Ah, that's what I have
to do." And it's pretty repeatable after
that. But it's also something you can
have a whole class to
buy a box of garbage bags. No big deal.
Right. But you know what I find the most
challenging part of that is to demo that
in front of the whole
class. They got rid of all
the overhead projectors. Right? That was
the perfect place to demo
it. So if two people held
polarizers, right, show that when you
cross them, it goes black and
open it up. And with those two
people holding those, another person
would come in and stretch the
polyethylene garbage bag and
then rotate it. And while it's not as
even as a polarizer, you can
definitely see all the changes
in the intensity on the screen. But they
got rid of that. And
document cameras don't quite cut it
because not enough light goes through.
And so it's a little harder to do. So I
wish they'd bring back
some overhead projectors just for that
demo. Those things were
great. The other one I really like
is how you can have students feel
entropy. And that of course is
the rubber band one. Have you
seen that one, Tim? Oh, yeah. And so it
turns out that your
forehead is very, very sensitive to
temperature. So if you take a rubber
band, you pull it, stretch
it. And then you know, if you
pull it really quickly and put it to your
head, it feels hotter. And
why is that? Because all those
elastomers are sliding along each other,
creating friction, and
it's getting heated, just dual
heating, right? But then if you take your
stretched out polymer and
you release it very quickly and
put it on your head, it is noticeably
cold. Cooler. Yeah. It's
colder than your ambient was.
And why is that? That's because all those
ordered chains have disordered
and entropy and temperature are
intrinsically related. And so you can
feel entropy. And what
I like about this one is every single
student gets to do the demo
themselves, which is ideal.
Unfortunately, with large classes, we
can't always do that. But
with rubber bands are so cheap,
you can pass them all around. Then you
see everybody pulling
these things and it's just
clicking it on the floor. And you know,
entropy is a hard concept to understand.
And hopefully this will give them some
insight into it. And the
very fact that polymers are
these big chains that are either ordered
or disordered, it should
work. And that's one that
almost always works. The other demo I
really like is when you
teach fatigue. So fatigue is
very statistical in nature. It's not
very, what's the word? It doesn't, oh,
there's a word for this.
It doesn't follow from first principles,
right? It's not
reproducible in the way that,
you know, the number of dislocations when
you strain something might be. And
it's, there is a lot of noise and it's
because the origin of fatigue
is a flaw. And you don't know
what the flaws are or how they're
distributed in the material.
So when you stress, even though
you're doing it well below the yield
stress, you're still accumulating defects
and accumulating and
changing internal flaws, cracks, all of
this stuff. So finally,
when you do it too much,
it catastrophically fails. And that's why
fatigue is so dangerous. So
little things like when, you
know, you teach mechanical properties,
you want students to
understand how to calculate an
appropriate safety factor. And it's
usually pretty easy. You
choose at least two, maybe 10,
and you just multiply, you know, the
expected maximum yield
strength and stress that you're
going to have on it and you're done. You
can't do that with fatigue because for
fatigue, you need to
understand the probabilistic nature of
how it fails. So those SN
curves, those are just the 50%
line curves. What really matters is how
all the data is collected
and how it distributes. So the
90th percentile, how far away from that
50% line is that? And
that's going to be different for
different materials, different processing
conditions, it's complicated. So to try
to illustrate that to
students, we give them paperclips and
make a quarter of the class
bend at 90 degrees and back,
that's one cycle, and they keep doing
that. And each person reports
their own number of cycles to
failure. Then the next quarter of the
class does 180 and back, 180 and back.
The next one does 270,
and the last group does 360. Well, the
360 group, of course, those
are going to fail much earlier.
But you're also going to get a much
bigger spread of the data. And when we
look at the raw data,
we can show the students using the class
collectively how much
spread in the data there is
and how that spread changes for each of
these different, well, we
call them loadings. It's
really not quite fatigue, but it's a
strained version of it.
And I think that's a really
valuable thing. And it helps them
understand that. The other demo we do,
it's gotten a little,
what's the word? It's what we used to do,
we don't do anymore, because
some people get embarrassed.
We used to do ask everybody to report
their weight in pounds. And
even though it was anonymous,
people got upset about it. So now we do
height. We ask everybody to
report their height in inches.
And then we show the height average and
the number average
difference of those sets to talk about
polymers with different lengths of
change, just to show them
that you get different results,
depending on how you count and why it
might be important. So
those kinds of demonstrations,
those are still participatory, they might
not be physical, but you're still
illustrating a point.
That's important. One demo I'd love to
do, I don't know if we can pull this off,
but it's the DaVinci demo. Do you know
which one that is? No,
what's that? So Leonardo DaVinci,
way back when he was alive, he did
experiments and he proved
that ropes that were longer
were weaker than ropes that were shorter.
And this usually
blows our students' mind,
you know, the ropes are the same. But of
course, it was because
of Weibull statistics.
And Weibull statistics is just weak link
theory. So the longer a rope is,
statistically, you're
going to have more flaws in the longer
rope, bigger flaws, leading to it
failing, because you'll have
a better chance of a weak link and a long
rope than a short rope.
And DaVinci did that. That's
pretty cool that he did that. And so it'd
be really fun if we had
like some really long ropes
and enough heavy weights to actually see
them break. It would
probably get expensive and take
a lot of space up. But it's kind of a
cool thing that he did that well before
Weibull did his Weibull
statistics. Yeah, that would be an
interesting one to try to turn into a
full class activity,
it'd be a matter of testing out different
types of ropes, different
amounts of weight, so on to
figure out what gives results that are
sort of messy enough, but
still work. And we kind of do
that with one of our projects we do in
our MyIntro class, because I
don't give exams, I have a lot
of time, and because I don't lecture, I
have a lot of time. So for
the part of the class where we
cover mechanical properties, my project
is to build a mechanical testing
instrument out of garbage,
you can't spend more than five bucks. And
so students are
constantly, you know, taking thread,
or taking a laffy toffee and clamping
things to them and looking how it
stresses or strains or
breaks. And it's a lot of fun. And that's
another way to have students
come up with their own demos
is a really fun way. And then of course,
they have to explain to
experts walking around the room,
why it happened. But it's just more
engagement, and it's more
physical. And again, it just
helps the student understand in a
different context than just reading a
book and doing homework
problems, how these phenomena actually
work, so that they can
use the concepts that we're
trying to teach them for whatever they do
in the future. So what other demos?
You've worked on a lot
of demos. I don't know if you have the
list I just gave you, but you can talk
about some of those.
Yeah, I suppose we should round out with
a couple of ceramics
oriented ones. So the first one that
comes to my mind, I love this. This is
also one that requires a
little bit of finesse. But it is
glass the conductor, I learned this one
from the ASM teachers camps,
actually, I've gotten several
demos from them over the years. And the
way you have this set up is
that you've got a light bulb,
and you have an open switch, essentially
in the circuit feeding the
light bulb, and you bridge
that switch with a glass rod. And of
course, the light bulb
doesn't light up because glass is an
insulator, allegedly. But what people
often forget is that
processing is really important.
And the the demo, what you do is you heat
the glass rod with a blowtorch.
And as it gets hot enough, the mobility
of the sodium ions in the
glass, so you do want to use
soda lime glass for this, it'll have a
higher density of sodium
ions in it, they get enough
mobility to where they can actually carry
enough current to light the
light bulb. And if you really
nail it just right, you can actually get
it to self sustain as well
where you take away the torch,
and the resistive heating from the
current passing through the glass will
keep the glass hot enough
to keep the mobility high enough that
current can keep flowing. And
the the ASM master teachers who
have done this a zillion times, I've seen
them keep one going for
something like 20 minutes before it
finally petered out. But yeah, conducting
electricity through
glass with the application
of just a little heat, that's always a
winner for me. Well, and it's also really
important to explain
to students that it's not just electrons
that transport charge,
right? Many things can transport
charge, it's a charge carrier, not an
electron. Some things are
holes that are the dominant
carrier. Some things are ions, like in
the example you just showed,
sometimes they're solitons,
you know, double bond flipping, that make
things move. So it's
the fact that the ideas of
conductivity and resistivity are very
general, and they span across many
different kinds of charge
carriers. And that's a really important
thing to get students to
understand. So it's great for them
understanding glasses, but it's also
great for them
understanding electrical properties.
Yep. What about the making copper for
malachite? That's another ceramics one.
Oh, yeah, rocks are ceramics after all.
There's this is one that is
so fun to do with students,
partly, of course, it involves smoke and
fire. And so that's
exciting. But it's a really great
illustration of this very, very old
process of turning rocks into
metal. This is, I don't know,
5000 years old technology at this point,
and still quite relevant
today. So how it's set up
is that you get a piece of charcoal, not
like a brick hat, but a
good lump hardwood charcoal
large piece. This is your carbon source.
And you can carve or drill a
well, you're making a crucible
right in the charcoal, and you load it up
with some flakes of
malachite. And you heat it. Again,
I use a torch. And as you heat it, you're
going through a series of
chemical reactions, where
you are making carbon monoxide and carbon
dioxide as you as you make
the charcoal react with the air.
And then you have the reactions between
the malachite, which I'm
trying to get this from
memory, I think it's a copper carbonate
hydrate, maybe. But you are you're
dehydrating the rock,
and you're also de carbonate thing.
That's not a word. But you're
pulling the carbonate out of
the rock, as it reacts with the, the
carbon monoxide, and the
oxygen in the air in this
hot air that you're making. And what you
end up with is nothing left
but the copper. And the way
it looks visually, it's hard to describe
this in words, but you
start with this green rock,
and you heat it, and it gets glowing hot.
And students are like,
Okay, is anything happening,
you're just making the rock glow because
it's hot. But then
after a couple minutes,
you take away the flame, and you let it
cool down. And it's
still red. And they're like,
Why is it red? Well, you tell me, you
took high school chemistry, what is a
color change indicate,
and they're like, Oh, chemical reaction.
So we go through this. And
then I pull out these little
red rocks that I've made, hit them with a
hammer, and they don't break. They're
ductile, they smoosh,
they smear. And so then it's just another
one of those, huh, I
thought ceramics were brittle,
what happened? And eventually someone is
like, Oh, it's red because
it's copper, you turned it into
a metal. Yeah. So wonderful demo. Love
that. I learned that from
Kevin Jones actually props to
him for being my inspiration on that one.
But yeah, I love that for
sure. I even like if you
watch carefully, once it turns to copper,
the flame from the
blowtorch, you start seeing little
bits of green green, because you may
remember from your inorganic chemistry
lab course, that the color
of the gases tell you something about
what materials are
there. So it's kind of cool.
Anyway, there are tons more demos. I
think we're out of time. I
just realized this. We're at the
45 minute mark. Oh, my goodness. So talk
about demos all day, but I
won't, we should wrap it up.
So we'll wrap this up and let me play our
outro music. So with
that, thanks for joining us.
And we'll see you next
time. See you next time.
Creators and Guests
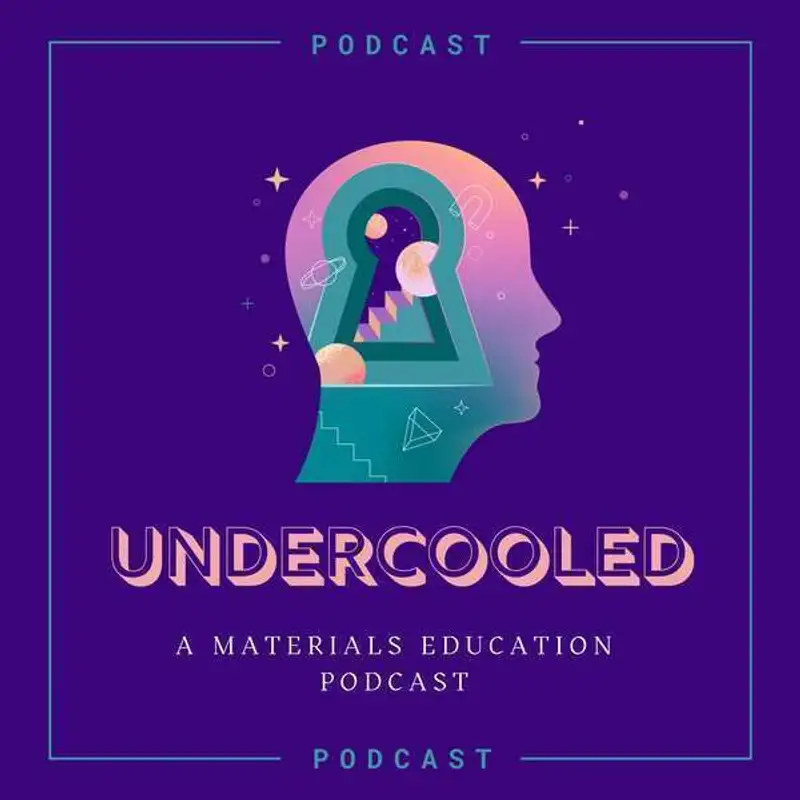